Preparation of cell
No special apparatus is needed for the cell. A 500 ml glass
food jar with a plastic cap is perfectly suitable. These are present as waste in
most households (e.g. from sauces, marmalade, or pickled gherkins).
Take approximately 100 grams of sodium bromide and add 300
warm water. The water can be plain tap water. Dissolve all of the sodium
bromide. The result is a little bit more than 300 ml of colorless liquid.
Drill or cut two holes in the cap of the jar, such that two
graphite rods can be pushed through these holes. No tight fit is needed, the
cell will be operated such that hardly any fumes are produced.
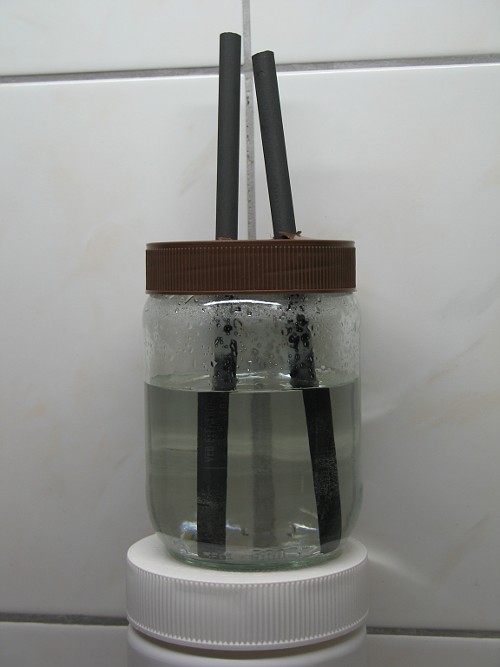
The cell as displayed can be used, but the result is far from optimal. After an
initial nice start the efficiency of the cell drops sharply and at the end its
efficiency almost drops to 0. Bromine is produced at the anode and in the cell
liquor this is converted to bromate, but at the cathode a large part of the
bromate is reduced back to bromide. If a few 100's of mg of hexavalent chromium
is added, then the cell works much better.
Dissolve
half a gram of potassium chromate or dichromate in a few ml of water and add
this to the cell liquid (sodium (di)chromate can also be used). This is the only
non-OTC compound, which may be hard to obtain. Making a few 100's of mg of this
in an impure state is not that difficult though (e.g. from pottery grade green
chromium oxide, or from chrome alum and hydrogen peroxide).
Wrap the
graphite rods above the cap of the jar in paper or plastic and fix them, such
that the cell becomes mechanically stable and no accidental short circuit can
occur when the jar is carefully moved around. How this is done is not really
important. In this setup some toilet paper was wrapped around the rods and some
paper was wrapped around the total of rods and paper and crocodile clamps were
used to fix the whole construction (see further below on pictures of the cell).
Preparation of circuit
The cell should not be connected directly to a constant
voltage power supply. Using such a supply leads to fairly strong variation in
the cell current during operation of the cell. For this experiment it is
important that the cell current is fairly constant. A nice value for the current
is somewhere between 1 and 2 A. With such a current the cell needs to be
operated for one or two days. In order to achieve a fairly constant current, a
series resistor must be used and the following circuit must be created.
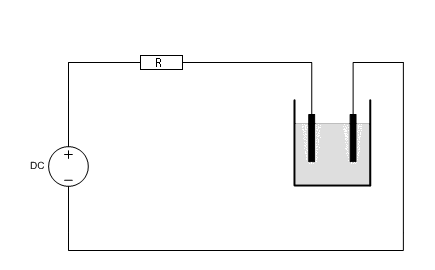
The DC power supply can be anything from 12 to 15 volts. The
resistor R can be anything from 5Ω to 10Ω. The resistor must be a big power
resistor, it becomes VERY hot. Use 25 Watt power resistors and if a single
resistor becomes too hot, then use a series connection of multiple resistors,
such that the heat is divided over multiple resistors.
The practical implementation of this circuit is shown below:
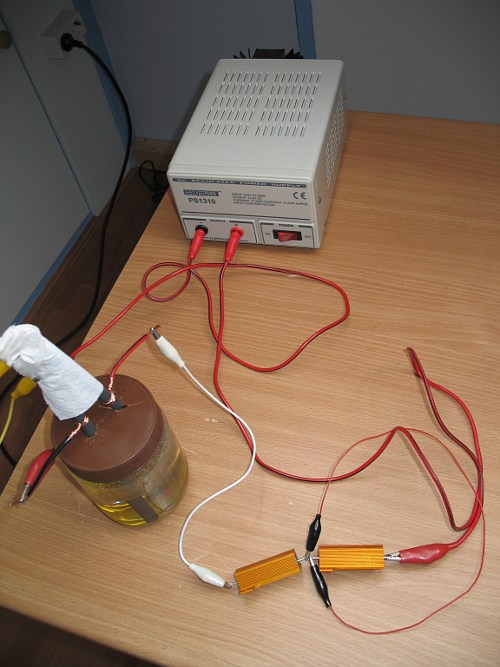
The power supply is a 13.8 V power supply and the resistors
are two pieces of 3.9Ω switched in series, so the total resistance is 7.8Ω.
There is nothing special about this value of 7.8Ω. These two 3.9Ω resistors just
happened to be available in my home lab. If resistors must be purchased for this
experiment, then it is suggested to buy 8 pieces of 1Ω/10W and connect all of
these in series. Such ceramic power resistors are cheap and will not cost
more than a few tens of cents per piece.
Keep in mind that during operation of the cell these resistors will become VERY
hot, even if 8 resistors are put in series!
Operation of circuit
Now, the cell must be operated for a certain amount of time.
Switch on the cell and allow it to run for an hour or so, such that it
stabilizes. After that hour measure the voltage across the resistor or series
connection of resistors. Compute the current from the measured voltage and the
total resistance. In my particular case, the measured voltage was 9.5 volts and
the total resistance was 7.8Ω. This means that the cell runs at 1.2 A.
The cell must operate for a certain amount of time, but not
for too long. In theory, the total running time of the cell should be as
follows:
T = F*M/I
Here F is Faraday's constant which is 96485 C/mol
M is the number of moles of sodium bromide. When exactly 100
grams of NaBr were used, then M is 100/(22.99+79.90) = 0.972.
I is the cell current. In my particular cell this is 1.2 A.
So, the total running time for my cell should be
96485*0.972/1.2 = 78153 seconds. This is almost 22 hours.
In practice, the cell efficiency is not 100%, due to back
reduction at the cathode. Without added hexavalent chromium the cell efficiency
is very low (maybe just a few percents), but with added hexavalent chromium the
cell efficiency usually is better than ⅔.
Overrunning the cell leads to losses, but underrunning the
cell leads to much larger losses. If the cell is overrun for 50%, then still 90%
of bromide is converted to bromine. Even if the cell is overrun for 100% (twice
as much oxidation as optimal) still 80% of bromide is converted to bromine. Even
small underrunning leads to catastrophic losses. So, just to be sure that the
cell is not underrun, the cell is allowed to run for 1.5 times the theoretically
computed time. So, for any practical purpose use the following formula for the
cell running time:
T = 1.5 * F * M / I
In my particular setup, the cell running time was 33 hours.
After a few hours of running, the cell liquor becomes turbid
and black crud collects at the bottom, due to loss of graphite from the anode.
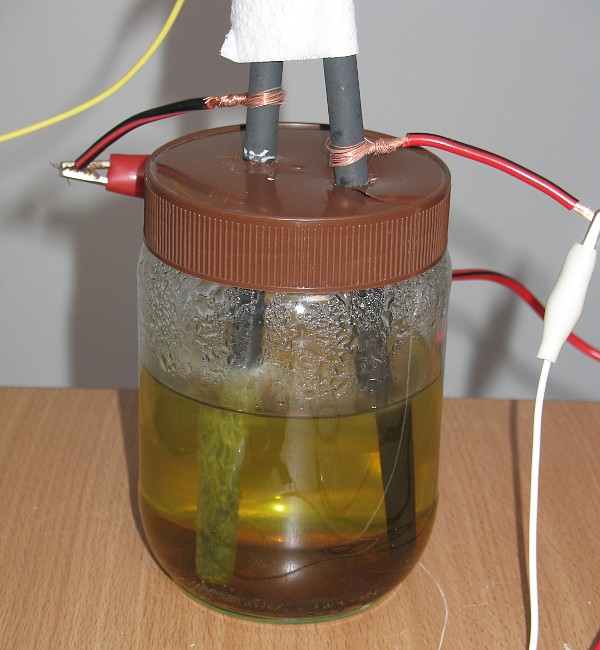
At the end of the running time, the liquid has become very
dark and a lot of black particles are floating around in the liquid.
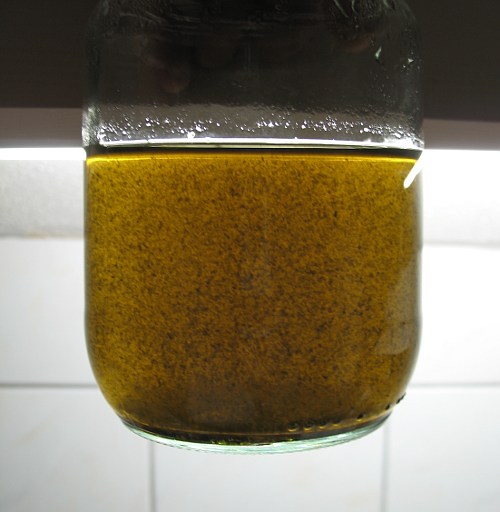
During the running time of the cell the liquid only becomes
luke warm and hardly any bad fumes are produced. This cell can be operated
inside without any problem as long as the cell current is not much more than 1
A.
Creation of bromine
After running the cell for sufficient time (see above) the
liquid ideally contains 1 bromate ion for each 5 bromide ions. As indicated
above, the cell is allowed to run for a longer time than theory predicts and
usually it contains a little more bromate than the ideal ratio of 1 : 5. This,
however, is not a real issue, it only leads to marginal losses.
Bromine now can be created, simply by adding acid. An acid,
which is available OTC and which is sufficiently strong is so-called pH-minus
for swimming pools. This is technical grade sodium bisulfate.
For each gram of sodium bromide take 1.5 grams of sodium
bisulfate. In my particular experiment with 100 gram of sodium bromide, 150
grams of sodium bisulfate was used. This material was put in another jar.
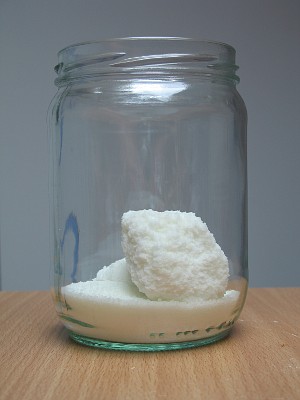
Dissolve the sodium bisulfate in as little as possible of tap
water. In my particular experiment, almost 300 ml of solution was obtained.
Filter
the contents of the cell, such that most of the graphite crud is left behind in
the filter. The resulting liquid is fairly clear.
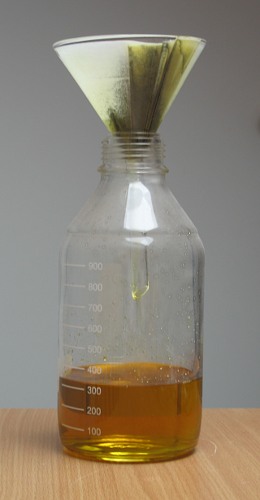
Pour the
solution of sodium bisulfate in the bottle with the filtered cell liquid. Do
this outside! Lots of vapor of bromine will be produced in this step. Do not
pour the solution of sodium bisulfate in the liquid at once but do this in three
steps of appr. 100 ml. After each addition swirl the bottle with liquid, such
that there is good mixing. The liquid heats up in this reaction, but if the
addition is not done too fast, then the liquid will not become hot. After adding
all solution of sodium bisulfate the liquid has become very dark and turbid, and
there also is very dark vapor of bromine above the liquid. Do not open the
bottle at this stage!
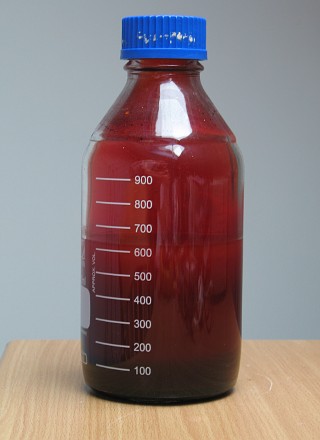
Allow
this bottle with liquid and vapor to stand in a cool place. A good option is to
put it in a bucket full of cold tap water for one hour. Lots of bromine droplets
will collect at the glass above the liquid and a layer of bromine will settle at
the bottom. After the hour of cooling, carefully swirl the liquid such that all
droplets of bromine, which collect at the glass in the upper part of the bottle
also are immersed in the liquid.
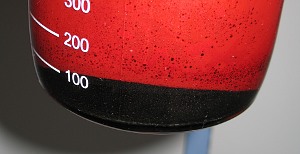
The pictures above show the bromine, settled at the bottom
and the much lighter liquid above the bromine.
Finally,
using a glass funnel, pour the liquid from the bottle, but keep the last
100 ml (which also holds the bromine). Pour the last 100 ml of liquid in a small
bottle of 100 ml and use a pipette to extract the bromine from the bottom.
Alternatively, pour the last 100 ml into a separating funnel and use that to
separate the bromine from the other liquid. This final step must be done
outside!
The final product is appr. 20 ml of bromine. This is
displayed at the top of this webpage.
The bromine can be bottled like this, but it is better if it is dried and
further purified. Drying and further purification, however, requires
concentrated sulphuric acid and a distillation setup. For most experiments and
many syntheses this crude bromine is perfectly suitable.
Total yield in this experiment is appr. 70% of all bromine. The remaining part
is in the 600 ml of liquid and in the vapor above that.
Discussion of results
This synthesis of bromine is based on a very simple
principle. Ideally for each bromide ion one electron is taken from the system,
such that all bromide is converted to bromine. In practice, this is done through
an intermediate step of formation of bromate.
In the electrolysis cell the following reactions occur:
At anode: 2Br– – 2e →
Br2
At cathode: 2H2O + 2e →
2OH– + H2
The hydrogen escapes as gas. The bromine dissolves in the
liquid and reacts with the hydroxide, formed at the cathode as well:
3Br2 + 6OH– →
5Br– + BrO3– + 3H2O
The reaction uses up exactly all bromine and hydroxide and
the liquid does not become alkaline, nor does hardly any bromine vapor escape
from the liquid.
For each 6 electrons, produced at the cathode (and absorbed
at the same time at the anode), one bromate ion is produced. Ideally, after the
electrolysis, 1/6 of all
bromide is converted to bromate and the number of electrons needed for that is
exactly the number of bromide ions in the cell when it is started. So, if 1 mole
of NaBr is used initially, then 1 mole of electrons must be passed through the
cell to convert 1/6 of
all bromide to bromate.
Current efficiency
In an ideal cell, the above reactions are the only ones which
occur. The net effect of these reactions is that for each mole of electrons
supplied by the power supply 1/6
mole of bromate ion is produced and three molecules of hydrogen. If only these
reactions occur, the cell efficiency is said to be 100%.
In a practical cell, there is back reduction of bromate at the cathode.
Bromate again is converted to bromide and hydroxide is produced as well. This
side reaction occurs instead of production of hydrogen gas and hydroxide ions.
When this occurs, then the cell efficiency becomes lower. A lower cell
efficiency means that less bromate is produced than what is expected on the
basis of theory.
Production of bromine, effect of overrunning and
underrunning the cell
The step of making bromine is based on the reaction between
bromide and bromate in acidic solution:
5Br– + BrO3–
+ 6H+ →
3Br2 + 3H2O
If exactly 1/6
of all bromide is converted to bromate, then all bromine in the liquid will end
up as elemental Br2 when sufficient acid is added. Now suppose that
there is a deviation from the ideal amount of bromate and that the total part of
bromate is (1+ε)/6.
For positive ε the cell is overrun (too much bromate is
formed). A cell, which is overrun for 50% has ε equal to ½. An overrun cell is
not really bad, even very strong overrunning like 50% or even 100% only leads to
moderate loss of bromine. The total amount of bromide is 1–(1+ε)/6 =
5/6×(1-ε/5). The bromide is
the limiting reactant for positive ε and the ideal amount is
5/6 part. With positive ε the
percentage of loss of bromine is only ε/5. So, for a cell which is overrun by
50% the loss only is 10% and for a cell which is overrun for 100% still the loss
only is 20%.
If on the other hand the value of ε is negative, then the
bromate is the limiting reactant. In that case, the relative loss is equal to ε.
I.e. for a cell, which is only underrun for 10% there also is 10% loss of
bromine and for a cell, which is underrun for 50% half of the bromine is lost.
There also is another undesirable side effect when the cell is underrun. If not
all bromide is used up, then the bromine is kept in solution and does not
precipitate. Bromine dissolves quite well in a solution, which is rich in
bromide:
Br2 + Br–
↔
Br3– This equilibrium is
quite strongly to the right. Bromine really dissolves quite well when bromide is
present as well. In a cell, which is underrun by a factor ε, there is a relative
loss of ε in terms of bromine production, but all non-converted bromine is
present as bromide, holding back another 2ε of bromine molecules. So, in
practice nearly 3ε is lost when precipitated bromine is isolated and bromine,
dissolved in the large amount of water is not recovered. Only by means of
leading air through the solution and condensing the bromine, taken with the air
the 2ε part of the bromine can be taken out of the solution. So, underrunning
the cell is very bad in terms of yield. Underrunning only 10% may lead to up to
30% additional loss, while overrunning 10% only leads to 2% additional loss. So,
for this reason it is really important to assure that the cell is not underrun
and take the slight loss of overrunning the cell for granted. |